- Details
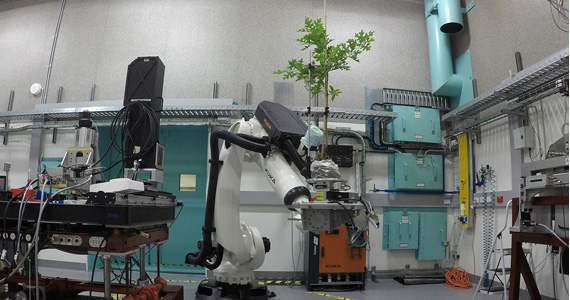
Researchers are using advanced imaging technologies similar to those used in hospitals, including micro-computed tomography on the Imaging and Medical beamline (IMBL) at the Australian Synchrotron, to determine how vulnerable our trees are to drought and heatwaves.
A new scientific review published In Nature outlines progress towards understanding the likely risks from droughts and heatwaves that combine to kill millions of trees around the world with spectacular effects on the environment.
Recent drought and heatwave conditions in northern Australia have killed more than 7000ha of mangrove forests, leaving these essential ecosystems stark, grey skeletons of trees. In California, the prolonged drought period has killed more than 100 million trees that increase the intensity of wildfires and impact on the region’s beauty, tourism and environmental health.
Dead trees, of course, cannot store carbon out of the air and the enormous numbers of dead trees release large quantities of stored carbon back into the air as they are burned or decay, further amplifying the effects of rising carbon dioxide.
In this research review released by Western Sydney University’s Hawkesbury Institute for the Environment and its international research team, scientists are using advanced technologies such as Magnetic Resonance Imaging (MRI ) to peer into living trees and map how increasing dehydration of the trees results in the progressive dieback of leaves and branches, eventually causing the trees to be unable to move water through the stems. As the trees weaken, they become susceptible to insect and disease attacks which ultimately can kill the tree outright.
“Access to imaging facilities such as the Australian Synchrotron are essential for conserving our environments and play a crucial role in ensuring we are ahead of a rapidly changing world climate”.
“Using our imaging and medical beamline for this study demonstrates the capacity of this instrument to provide deep, detailed information on how trees transport water and nutrients from roots to leaves. The IMBL is used to 3D image a wide range of samples from fossils to industrial materials to human tissue, but in this instance, we were providing insights into the impact of dehydration on plant physiology ” said Principal Instrument Scientist Dr Daniel Hausermann.
“Climatic changes are expected to increase the frequency and intensity of droughts and the combination of heat and dry really stresses even the hardiest trees”, explains Associate Professor Brendan Choat, lead author on the review.
“If we can work out, using non-invasive methods like MRIs, the point at which trees become too dehydrated to survive then we can establish a dataset that can be used in the field to tell if a tree is reaching the point of no return”, he explains.
Trees are only able to move water to the tops of their branches if there is enough water in the soil and if they can preserve the column of water in their stems without bubbles or breakages. Most trees are able to maintain this flow of water but extreme demand for water during heat coupled with increasing soil dryness can mean that the flow can be interrupted. This can result in the death of leaves, then branches and ultimately the whole tree.
“Knowing the limits of individual tree species puts us in a good position to understand how vulnerable trees are to death”, says Assoc Prof Choat.
Content provided by the University of Western Sydney and adapted. https://www.westernsydney.edu.au/newscentre/news_centre/more_news_stories/tree_mortality_nature
Nature Paper: https://www.nature.com/articles/s41586-018-0240-x
- Details
ANSTO has contributed to research led by the University of Sydney, involving doping transition metals in a polymorph of bismuth oxide in a search for more structural stability.
Cubic high-temperature polymorph of bismuth oxide, δ-Bi2O3, is the best known oxide ionic conductor but its narrow stability range (729 - 817 °C), which is close to its melting temperature of 817 °C precludes its practical use.
A large collaboration, led by Professor Chris Ling and Dr Julia Wind (as part of her PhD) from the University of Sydney involving researchers from ANSTO and two other universities, has achieved the design and understanding of the complex crystal structure and chemistry behind a commensurate structure within the fast-ion conducting stabilised bismuth oxide, co-doped with chromium and niobium, Bi23CrNb3O45.
The study was published in the Chemistry of Materials.
Dr Zhaoming Zhang from ANSTO provided her expertise in X-ray absorption spectroscopy to the study.
Low and high energy X-ray absorption near edge structure (XANES) data were obtained from the soft X-ray (SXR) and X-ray absorption spectroscopy (XAS) beamlines at the Australian Synchrotron respectively, and medium energy XANES data from the ‘Tender’ beamline at the National Synchrotron Radiation Research Center (NSRRC) in Taiwan, which helped to clarify details of the complex superstructure, in conjunction with neutron, synchrotron X-ray and electron diffraction, high resolution transmission electron microscopy and ab initio calculations.
“Spectroscopy is very important in this case because of the variety of possible oxidation states and local structures for the transition metal dopants,” said Zhang.
“XANES spectra not only gives you information on the oxidation state of the metal dopants but also the local coordination and site geometry around them, which was very informative for this investigation.”
The commensurate modulation was confirmed by selected area electron diffraction (SAED) patterns. (See image below)
The researchers had sought to find a truly commensurate version of the incommensurate Type II phase of bismuth oxide doped with mixed valence transition metals, in which a 3 X 3 X 3 supercell structure was ‘locked in’.
In a "normal" crystal, the crystal is built by repeating the unit cell by translation along the 3 directions of space.
For modulated structures, the periodicity is in more than three dimensions. If the ratio of the modulation period to the unit cell length is rational then the modulation is called ‘commensurate’, otherwise it becomes ‘incommensurate’.
Because the modulation vector ε varied with both the type of transition metal dopants and the bismuth to transition metal ratio, the investigators had two independent variables to chemically tune the composition in search of a commensurate ε = 1/3 structure, which was found in Bi23CrNb3O45.
“Interpreting the spectroscopy data was somewhat challenging because of the complexity of the superstructure. The results not only confirmed the presence of hexavalent chromium and pentavalent niobium in Bi23CrNb3O45, but also informed us that each niobium is surrounded by six oxygens and chromium by four oxygens,” said Zhang.
In the superstructure, the oxygens around transition metals are ordered locally forming tetrahedral clusters of NbO6 octahedra and isolated CrO4 tetrahedra, separating relatively disordered fluorite-type regions that facilitate the high oxide-ionic conduction. (See crystal structure above)
“Although doping of bismuth oxide with rare earth metals exhibits higher conductivity, the transition metal doped bismuth oxides may offer better long term stability,” said Zhang.
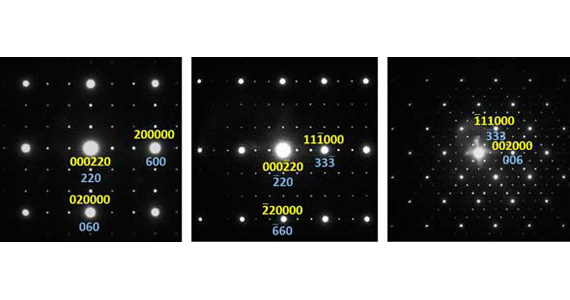
While this was a fundamental study, bismuth oxide is of considerable interest as a material because its mobile oxygen defects can act as carriers of charge in ionic devices, such as solid oxide fuel cells.
Collaborating organisations included the University of Sydney, ANSTO, the University of South Carolina in the US and the Australian National University. Neutron and synchrotron X-ray diffraction data were collected on the Echidna instrument at the Australian Centre for Neutron Scattering (ACNS) and the powder diffraction (PD) beamline at the Australian Synchrotron, with the assistance of Drs Max Avdeev and Justin Kimpton respectively. Drs Peter Kappen and Lars Thomsen assisted with the XANES experiments on the XAS and SXR beamlines at the Australian Synchrotron.
A new, medium energy XAS beamline is being planned at the Australian Synchrotron as part of the Br—GHT initiative. “Once it is constructed, we will no longer need to travel to Taiwan for medium energy XANES experiments,” said Zhang
- Details
Melbourne scientists have taken a significant step toward developing a new vaccine for malaria, revealing
for the first time an ‘atomic-scale’ blueprint of how the parasite invades human cells.
Using the Nobel Prize-winning technology cryo-EM (cryo-electron microscopy), the researchers mapped the
previously hidden first contact between Plasmodium vivax malaria parasites and young red blood cells they
invade to begin the parasites’ spread throughout the body.
The MX2 beamline and SAXS/WAXS beamline were also used by the investigators in this study.
The discovery was published in Nature on 28 June 2018.
Associate Professor Wai-Hong Tham and Dr Jakub Gruszczyk from Melbourne’s Walter and Eliza Hall
Institute – in collaboration with Dr Rick Huang and Dr Zhiheng Yu at the Howard Hughes Medical Institute
(US) – solved the mystery of the molecular machinery the parasite uses to latch on to red blood cells.
This essential step in the malaria lifecycle is the beginning of the classical symptoms associated with
malaria – fever, chills, malaise, diarrhoea and vomiting – which can last weeks or even longer.
Cryo-EM provides vaccine key
Earlier this year, the team discovered P. vivax parasites use the human transferrin receptor to gain access to
red blood cells, a study they published in Science. Now, with the aid of revolutionary cryo-EM technology,
Associate Professor Tham said the team was able to overcome previous technical challenges to visualise
the interaction at an atomic level.
“We’ve now mapped, down to the atomic level, exactly how the parasite interacts with the human transferrin
receptor,” Associate Professor Tham said.
“This is critical for taking our original finding to the next stage – developing potential new antimalarial drugs
and vaccines. Cryo-EM is really opening doors for researchers to visualise structures that were previously
too large and complex to ‘solve’ before.”
P. vivax is the most widespread malaria parasite worldwide, and the predominant cause of malaria in the
vast majority of countries outside Africa. Because of its propensity to ‘hide’ undetected by the immune
system in a person’s liver, it is also the number one parasite responsible for recurrent malaria infections.
Guided by the 3D map, Associate Professor Tham said the team was able to tease out the precise details of
the parasite-host interaction, identifying its most vulnerable spots.
“It’s basically a design challenge. P. vivax parasites are incredibly diverse – which is challenging for vaccine
development. We have now identified the molecular machinery that would be the best target for an
antimalarial vaccine effective against the widest range of P. vivax parasites,” she said.
“With this unprecedented level of detail, we can now begin to design new therapies that specifically target
and disrupt the parasite’s invasion machinery, preventing malaria parasites from hijacking human red blood
cells to spread through the blood and, ultimately, be transmitted to others.”
Exploiting weak spots
Dr Gruszczyk said the team also ‘solved’ how antimalarial antibodies bind to and block P. vivax parasites to
stop them from invading red blood cells, using X-ray crystallography on the MX2 beamline at the Australian Synchrotron.
“With this crystal map, we have identified additional ‘weak spots’ that could be exploited as therapeutic
targets. The information allows us to go back to the parasite and pull out the part of the protein that will
make the best possible vaccine,” Dr Gruszczyk said.
The research was supported by the Australian Research Council, Speedy Innovation Grant, National Health
and Medical Research Council, Howard Hughes Medical Institute, Wellcome Trust, Drakensberg Trust and
the Victorian Government.
Content provided by Walter and Eliza Hall Institute and adapted slightly